Step by Step: Building the Toolkit to Understand the Brain
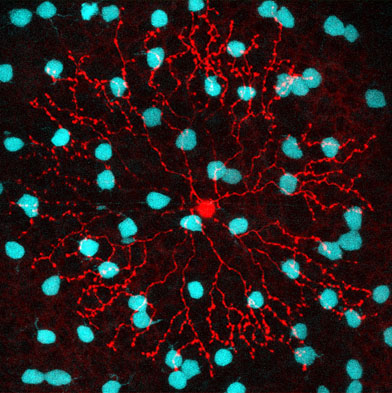
Among the areas of neuroscience in which Neuroscience Institute scientists aim to make the greatest contribution is figuring out how the brain’s neural circuits process sensory data into perceptions and behavior.
To say this may be biology’s ultimate challenge is stating the obvious. How does one parse out the roles of millions of neurons, connected in unseen ways within our most vital organ? And how does this network of cells make the calculations to figure out what something is, how important it is, how we should respond, and how long we should retain the information? All at incredible speeds? And learn to do all this from birth, by self-assembling this network?
According to Wei Wei, PhD, one of the best places to study neural circuits is the retina, the camera-like organ in the back of our eye which so precisely captures light, extracts relevant information on motion, contrast, and color, and sends these on to higher brain centers. Not only are the circuits in the retina much more accessible than those on the inside of the brain (as you know if you’ve ever had a retinal exam), they also arise from a better-defined variety of well-described cells.
So Wei and her lab are using the retina as a testbed, a shortcut to get at the general principles that guide the function of larger neural networks. For despite the fact that neural circuits are complex and diverse, it appears all are built up from the same types of smaller modules which register and transmit signals in predictable ways, in many different species. Understanding how these basic elements manage visual signals in the retina will allow us to analogize about the more remote and inaccessible circuits in the brain.
But even assessing these retinal circuit is complicated. How do you observe the neurons and the dynamic connections between them in living, behaving mammals?
Wei and colleagues have become experts in doing what scientists have done for centuries—and mankind in general does so well—devising new tools, or adapting older ones to get a challenging job done. If Wei could find a way to observe the reactions and operations of retinal cells in action, her team could develop some important clues to how larger neural networks perform—and reduce the search space for answers on how the entire nervous system is put together.
One tool—used by biologists for fifty years—is fluorescent proteins. With these little molecules, Wei and her lab can tag individual cells, just as teenagers “tag” train cars so they can follow their markings all over the city or countryside. But unlike the names or symbols painted on train cars, fluorescent proteins are visible only when excited by light—which is also, of course the very stimulus that invokes the response from retinal neurons. So if you use a visible-light microscope to observe the neurons, you are, in fact, creating an observer effect, where you are altering the very state you want to measure.
So Wei’s lab adapted a second tool to circumvent this problem. Two-photon microscopes excite fluorescent proteins with light of longer wavelengths—in the infrared range—to “see” the fluorescing proteins. Not only is the infrared light invisible to the retinal cells—so they won’t react—but the fast, focused bursts of light suppress the image backgrounds, localize the focus, and increase the resolution of the signal images.
Although two-photon microscopy is technically quite sophisticated, the theory for its use was actually developed before protein tagging. Maria Goeppert Mayer, an American theoretical physicist born in Katowice, now in Poland, described the approach in her 1930 PhD dissertation, which she earned in the lab of the famous Max Born. Goeppert Mayer, who won the 1963 Nobel Prize in Physics for proposing the nuclear shell model of the atomic nucleus, was named a “voluntary associate professor of physics” at the University of Chicago during the Manhattan Project. (Although UChicago granted her the title of professor—unlike Johns Hopkins and Columbia, where she held lower-level, “assistant” positions as a favor to her physicist-husband, Joe Mayer—UChicago also didn’t pay her. The University of California, San Diego eventually did, but Goeppert Mayer died soon after.) Scientists at Cornell University finally patented two-photon microscopy in 1990.
This tiny slice of history makes it clear how progress in neuroscience depends on breakthroughs in other domains—especially physics and mathematics, Goeppert Mayer’s original field and one on which she drew heavily in constructing her models for the atom’s nuclear shell. That’s why the Neuroscience Institute was designed as a “big tent,” to bring physicists, mathematicians, neurobiologists, psychologists, and others together to make these breakthroughs more quickly.
By combining stimulation techniques common in vision research with two-photon microscopy, usually used to study brain tissues not sensitive to light, Wei’s lab can undertake many experiments to probe neural circuits once considered infeasible. She hopes that what she learns about synapses in retinal circuits can inform how the entire central nervous system connects, develops, and enables us to design tools—like the ones Wei is using so creatively to help us understand ourselves.
Elise Wachspress is a senior communications strategist for the University of Chicago Medicine & Biological Sciences Development.